Transcription activator-like effector nuclease
Transcription activator-like effector nucleases (TALEN) are restriction enzymes that can be engineered to cut specific sequences of DNA. They are made by fusing a TAL effector DNA-binding domain to a DNA cleavage domain (a nuclease which cuts DNA strands). Transcription activator-like effectors (TALEs) can be engineered to bind practically any desired DNA sequence, so when combined with a nuclease, DNA can be cut at specific locations.[1] The restriction enzymes can be introduced into cells, for use in gene editing or for genome editing in situ, a technique known as genome editing with engineered nucleases. Alongside zinc finger nucleases and CRISPR/Cas9, TALEN is a prominent tool in the field of genome editing.
TALE DNA-binding domain
TAL effectors are proteins that are secreted by Xanthomonas bacteria via their type III secretion system when they infect plants.[2] The DNA binding domain contains a repeated highly conserved 33–34 amino acid sequence with divergent 12th and 13th amino acids. These two positions, referred to as the Repeat Variable Diresidue (RVD), are highly variable and show a strong correlation with specific nucleotide recognition.[3][4] This straightforward relationship between amino acid sequence and DNA recognition has allowed for the engineering of specific DNA-binding domains by selecting a combination of repeat segments containing the appropriate RVDs.[1] Notably, slight changes in the RVD and the incorporation of "nonconventional" RVD sequences can improve targeting specificity.[5]
DNA cleavage domain
The non-specific DNA cleavage domain from the end of the FokI endonuclease can be used to construct hybrid nucleases that are active in a yeast assay.[6][7] These reagents are also active in plant cells[8][9] and in animal cells.[9][10][11][12] Initial TALEN studies used the wild-type FokI cleavage domain, but some subsequent TALEN studies[11][13][14] also used FokI cleavage domain variants with mutations designed to improve cleavage specificity[15][16] and cleavage activity.[17] The FokI domain functions as a dimer, requiring two constructs with unique DNA binding domains for sites in the target genome with proper orientation and spacing. Both the number of amino acid residues between the TALE DNA binding domain and the FokI cleavage domain and the number of bases between the two individual TALEN binding sites appear to be important parameters for achieving high levels of activity.[10][18]
Engineering TALEN constructs
The simple relationship between amino acid sequence and DNA recognition of the TALE binding domain allows for the efficient engineering of proteins. In this case, artificial gene synthesis is problematic because of improper annealing of the repetitive sequence found in the TALE binding domain.[19] One solution to this is to use a publicly available software program (DNAWorks) to calculate oligonucleotides suitable for assembly in a two step PCR oligonucleotide assembly followed by whole gene amplification. A number of modular assembly schemes for generating engineered TALE constructs have also been reported.[9][19][20][21][22][23] Both methods offer a systematic approach to engineering DNA binding domains that is conceptually similar to the modular assembly method for generating zinc finger DNA recognition domains.
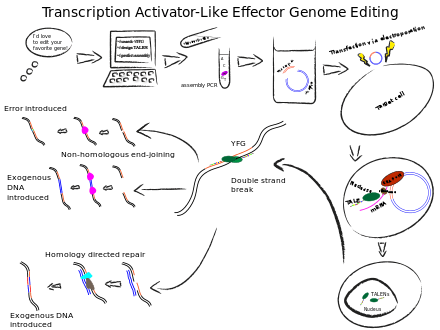
Transfection
Once the TALEN constructs have been assembled, they are inserted into plasmids; the target cells are then transfected with the plasmids, and the gene products are expressed and enter the nucleus to access the genome. Alternatively, TALEN constructs can be delivered to the cells as mRNAs, which removes the possibility of genomic integration of the TALEN-expressing protein. Using an mRNA vector can also dramatically increase the level of homology directed repair (HDR) and the success of introgression during gene editing.
Genome editing
Mechanisms
TALEN can be used to edit genomes by inducing double-strand breaks (DSB), which cells respond to with repair mechanisms.
Non-homologous end joining (NHEJ) directly ligates DNA from either side of a double-strand break where there is very little or no sequence overlap for annealing. This repair mechanism induces errors in the genome via indels (insertion or deletion), or chromosomal rearrangement; any such errors may render the gene products coded at that location non-functional.[10] Because this activity can vary depending on the species, cell type, target gene, and nuclease used, it should be monitored when designing new systems. A simple heteroduplex cleavage assay can be run which detects any difference between two alleles amplified by PCR. Cleavage products can be visualized on simple agarose gels or slab gel systems.
Alternatively, DNA can be introduced into a genome through NHEJ in the presence of exogenous double-stranded DNA fragments.[10]
Homology directed repair can also introduce foreign DNA at the DSB as the transfected double-stranded sequences are used as templates for the repair enzymes.[10]
Applications
TALEN has been used to efficiently modify plant genomes,[24] creating economically important food crops with favorable nutritional qualities.[25] They have also been harnessed to develop tools for the production of biofuels.[26] In addition, it has been used to engineer stably modified human embryonic stem cell and induced pluripotent stem cell (IPSCs) clones and human erythroid cell lines,[11][27] to generate knockout C. elegans,[12] knockout rats,[13] knockout mice,[28] and knockout zebrafish.[14][29] Moreover, the method can be used to generate knockin organisms. Wu et al.obtained a Sp110 knockin cattle using Talen nickases to induce increased resistance of tuberculosis.[30] This approach has also been used to generate knockin rats by TALEN mRNA microinjection in one-cell embryos.[31]
TALEN has also been utilized experimentally to correct the genetic errors that underlie disease.[32] For example, it has been used in vitro to correct the genetic defects that cause disorders such as sickle cell disease,[27][33] xeroderma pigmentosum,[34] and epidermolysis bullosa.[35] Recently, it was shown that TALEN can be used as tools to harness the immune system to fight cancers; TALEN-mediated targeting can generate T cells that are resistant to chemotherapeutic drugs and show anti-tumor activity.[36][37]
In theory, the genome-wide specificity of engineered TALEN fusions allows for correction of errors at individual genetic loci via homology-directed repair from a correct exogenous template.[32] In reality, however, the in situ application of TALEN is currently limited by the lack of an efficient delivery mechanism, unknown immunogenic factors, and uncertainty in the specificity of TALEN binding.[32]
Another emerging application of TALEN is its ability to combine with other genome engineering tools, such as meganucleases. The DNA binding region of a TAL effector can be combined with the cleavage domain of a meganuclease to create a hybrid architecture combining the ease of engineering and highly specific DNA binding activity of a TAL effector with the low site frequency and specificity of a meganuclease.[38]
In comparison to other genome editing techniques TALEN falls in the middle in terms of difficulty and cost. Unlike ZNFs, TALEN recognizes single nucleotides. It's far more straightforward to engineer interactions between TALEN DNA binding domains and their target nucleotides than it is to create interactions with ZNFs and their target nucleotide triplets.[39] On the other hand, CRISPR relies on ribonucleotide complex formation instead of protein/DNA recognition. gRNAs can target nearly any sequence in the genome and they can be cheaply produced, thus making CRISPR more efficient and less expensive than both TALEN and ZNF. TALEN is ultimately 200 times more expensive than CRISPR and takes several months more to perform.[40]
TAL effector nuclease precision
The off-target activity of an active nuclease may lead to unwanted double-strand breaks and may consequently yield chromosomal rearrangements and/or cell death. Studies have been carried out to compare the relative nuclease-associated toxicity of available technologies. Based on these studies [18] and the maximal theoretical distance between DNA binding and nuclease activity, TALEN constructs are believed to have the greatest precision of the currently available technologies.[41]
See also
References
- 1 2 Boch, Jens (February 2011). "TALEs of genome targeting". Nature Biotechnology. 29 (2): 135–6. doi:10.1038/nbt.1767. PMID 21301438.
- ↑ Boch J, Bonas U (September 2010). "XanthomonasAvrBs3 Family-Type III Effectors: Discovery and Function". Annual Review of Phytopathology. 48: 419–36. doi:10.1146/annurev-phyto-080508-081936. PMID 19400638.
- ↑ Boch, Jens; et al. (December 2009). "Breaking the Code of DNA Binding Specificity of TAL-Type III Effectors". Science. 326 (5959): 1509–12. Bibcode:2009Sci...326.1509B. doi:10.1126/science.1178811. PMID 19933107.
- ↑ Moscou, Matthew J.; Adam J. Bogdanove (December 2009). "A Simple Cipher Governs DNA Recognition by TAL Effectors". Science. 326 (5959): 1501. Bibcode:2009Sci...326.1501M. doi:10.1126/science.1178817. PMID 19933106.
- ↑ Juillerat, Alexandre; Pessereau, Coline; Dubois, Gwendoline; Guyot, Valérie; Maréchal, Alan; Valton, Julien; Daboussi, Fayza; Poirot, Laurent; Duclert, Aymeric (2015-01-01). "Optimized tuning of TALEN specificity using non-conventional RVDs". Scientific Reports. 5: 8150. doi:10.1038/srep08150. ISSN 2045-2322. PMC 4311247
. PMID 25632877.
- ↑ Christian, Michelle; et al. (October 2010). "Targeting DNA Double-Strand Breaks with TAL Effector Nucleases". Genetics. 186 (2): 757–61. doi:10.1534/genetics.110.120717. PMC 2942870
. PMID 20660643.
- ↑ Li, Ting; et al. (August 2010). "TAL nucleases (TALNs): hybrid proteins composed of TAL effectors and FokI DNA-cleavage domain". Nucleic Acids Research. 39: 1–14. doi:10.1093/nar/gkq704. PMC 3017587
. PMID 20699274.
- ↑ Mahfouz, Magdy M.; et al. (February 2010). "De novo-engineered transcription activator-like effector (TALE) hybrid nuclease with novel DNA binding specificity creates double-stran breaks". PNAS. 108 (6): 2623–8. Bibcode:2011PNAS..108.2623M. doi:10.1073/pnas.1019533108. PMC 3038751
. PMID 21262818.
- 1 2 3 Cermak, T.; Doyle, E. L.; Christian, M.; Wang, L.; Zhang, Y.; Schmidt, C.; Baller, J. A.; Somia, N. V.; Bogdanove, A. J.; Voytas, D. F. (2011). "Efficient design and assembly of custom TALEN and other TAL effector-based constructs for DNA targeting". Nucleic Acids Research. 39 (12): e82. doi:10.1093/nar/gkr218. PMC 3130291
. PMID 21493687.
- 1 2 3 4 5 Miller, Jeffrey; et al. (February 2011). "A TALE nuclease architecture for efficient genome editing". Nature Biotechnology. 29 (2): 143–8. doi:10.1038/nbt.1755. PMID 21179091.
- 1 2 3 Hockemeyer, D.; Wang, H.; Kiani, S.; Lai, C. S.; Gao, Q.; Cassady, J. P.; Cost, G. J.; Zhang, L.; Santiago, Y.; Miller, J. C.; Zeitler, B.; Cherone, J. M.; Meng, X.; Hinkley, S. J.; Rebar, E. J.; Gregory, P. D.; Urnov, F. D.; Jaenisch, R. (2011). "Genetic engineering of human pluripotent cells using TALE nucleases". Nature Biotechnology. 29 (8): 731–734. doi:10.1038/nbt.1927. PMC 3152587
. PMID 21738127.
- 1 2 Wood, A. J.; Lo, T. -W.; Zeitler, B.; Pickle, C. S.; Ralston, E. J.; Lee, A. H.; Amora, R.; Miller, J. C.; Leung, E.; Meng, X.; Zhang, L.; Rebar, E. J.; Gregory, P. D.; Urnov, F. D.; Meyer, B. J. (2011). "Targeted Genome Editing Across Species Using ZFNs and TALENs". Science. 333 (6040): 307. doi:10.1126/science.1207773. PMC 3489282
. PMID 21700836.
- 1 2 Tesson, L.; Usal, C.; Ménoret, S. V.; Leung, E.; Niles, B. J.; Remy, S. V.; Santiago, Y.; Vincent, A. I.; Meng, X.; Zhang, L.; Gregory, P. D.; Anegon, I.; Cost, G. J. (2011). "Knockout rats generated by embryo microinjection of TALENs". Nature Biotechnology. 29 (8): 695–696. doi:10.1038/nbt.1940. PMID 21822240.
- 1 2 Huang, P.; Xiao, A.; Zhou, M.; Zhu, Z.; Lin, S.; Zhang, B. (2011). "Heritable gene targeting in zebrafish using customized TALENs". Nature Biotechnology. 29 (8): 699–700. doi:10.1038/nbt.1939.
- ↑ Doyon, Y.; Vo, T. D.; Mendel, M. C.; Greenberg, S. G.; Wang, J.; Xia, D. F.; Miller, J. C.; Urnov, F. D.; Gregory, P. D.; Holmes, M. C. (2010). "Enhancing zinc-finger-nuclease activity with improved obligate heterodimeric architectures". Nature Methods. 8 (1): 74–79. doi:10.1038/nmeth.1539. PMID 21131970.
- ↑ Szczepek, M.; Brondani, V.; Büchel, J.; Serrano, L.; Segal, D. J.; Cathomen, T. (2007). "Structure-based redesign of the dimerization interface reduces the toxicity of zinc-finger nucleases". Nature Biotechnology. 25 (7): 786–793. doi:10.1038/nbt1317. PMID 17603476.
- ↑ Guo, J.; Gaj, T.; Barbas Iii, C. F. (2010). "Directed Evolution of an Enhanced and Highly Efficient FokI Cleavage Domain for Zinc Finger Nucleases". Journal of Molecular Biology. 400 (1): 96–107. doi:10.1016/j.jmb.2010.04.060. PMC 2885538
. PMID 20447404.
- 1 2 Mussolino, C.; Morbitzer, R.; Lutge, F.; Dannemann, N.; Lahaye, T.; Cathomen, T. (2011). "A novel TALE nuclease scaffold enables high genome editing activity in combination with low toxicity". Nucleic Acids Research. 39: 9283–9293. doi:10.1093/nar/gkr597.
- 1 2 Zhang, Feng; et al. (February 2011). "Efficient construction of sequence-specific TAL effectors for modulating mammalian transcription". Nature Biotechnology. 29 (2): 149–53. doi:10.1038/nbt.1775. PMC 3084533
. PMID 21248753.
- ↑ Morbitzer, R.; Elsaesser, J.; Hausner, J.; Lahaye, T. (2011). "Assembly of custom TALE-type DNA binding domains by modular cloning". Nucleic Acids Research. 39: 5790–5799. doi:10.1093/nar/gkr151.
- ↑ Li, T.; Huang, S.; Zhao, X.; Wright, D. A.; Carpenter, S.; Spalding, M. H.; Weeks, D. P.; Yang, B. (2011). "Modularly assembled designer TAL effector nucleases for targeted gene knockout and gene replacement in eukaryotes". Nucleic Acids Research. 39: 6315–6325. doi:10.1093/nar/gkr188.
- ↑ Geiβler, R.; Scholze, H.; Hahn, S.; Streubel, J.; Bonas, U.; Behrens, S. E.; Boch, J. (2011). Shiu, Shin-Han, ed. "Transcriptional Activators of Human Genes with Programmable DNA-Specificity". PLoS ONE. 6 (5): e19509. doi:10.1371/journal.pone.0019509. PMC 3098229
. PMID 21625585.
- ↑ Weber, E.; Gruetzner, R.; Werner, S.; Engler, C.; Marillonnet, S. (2011). Bendahmane, Mohammed, ed. "Assembly of Designer TAL Effectors by Golden Gate Cloning". PLoS ONE. 6 (5): e19722. doi:10.1371/journal.pone.0019722.
- ↑ Zhang, Yong; Zhang, Feng; Li, Xiaohong; Baller, Joshua A.; Qi, Yiping; Starker, Colby G.; Bogdanove, Adam J.; Voytas, Daniel F. (2013-01-01). "Transcription activator-like effector nucleases enable efficient plant genome engineering". Plant Physiology. 161 (1): 20–27. doi:10.1104/pp.112.205179. ISSN 1532-2548. PMC 3532252
. PMID 23124327.
- ↑ Haun, William; Coffman, Andrew; Clasen, Benjamin M.; Demorest, Zachary L.; Lowy, Anita; Ray, Erin; Retterath, Adam; Stoddard, Thomas; Juillerat, Alexandre (2014-09-01). "Improved soybean oil quality by targeted mutagenesis of the fatty acid desaturase 2 gene family". Plant Biotechnology Journal. 12 (7): 934–940. doi:10.1111/pbi.12201. ISSN 1467-7652. PMID 24851712.
- ↑ Daboussi, Fayza; Leduc, Sophie; Maréchal, Alan; Dubois, Gwendoline; Guyot, Valérie; Perez-Michaut, Christophe; Amato, Alberto; Falciatore, Angela; Juillerat, Alexandre (2014-01-01). "Genome engineering empowers the diatom Phaeodactylum tricornutum for biotechnology". Nature Communications. 5: 3831. doi:10.1038/ncomms4831. ISSN 2041-1723. PMID 24871200.
- 1 2 http://www.nature.com/ncomms/2015/150514/ncomms8085/full/ncomms8085.html
- ↑ Davies, B; Davies, G; Preece, C; Puliyadi, R; Szumska, D; et al. (2013). "Site Specific Mutation of the Zic2 Locus by Microinjection of TALEN mRNA in Mouse CD1, C3H and C57BL/6J Oocytes". PLOS ONE. 8: e60216. doi:10.1371/journal.pone.0060216.
- ↑ Sander; et al. "Targeted gene disruption in somatic zebrafish cells using engineered TALENs". Nature Biotechnology. 29: 697–98. doi:10.1038/nbt.1934.
- ↑ Wu; et al. "TALE nickase-mediated SP110 knockin endows cattle with increased resistance to tuberculosis". Proceedings of the National Academy of Sciences of the United States of America. 112: E1530–E1539. doi:10.1073/pnas.1421587112.
- ↑ Ponce; de León, V; et al. (2014). ", Generation of TALEN-mediated GRdim knock-in rats by homologous recombination". PLOS ONE. 9 (2): e88146. doi:10.1371/journal.pone.0088146.
- 1 2 3 Carlson, D. F. & Fahrenkrug, S. C. (2012). "Targeting DNA with fingers and TALENs" (PDF). Molecular Therapy- Nucleic Acids. 1 (3): e3. doi:10.1038/mtna.2011.5. PMC 3381595
. PMID 23344620. Retrieved November 11, 2014.
- ↑ Ramalingam, S. (2014). "TALEN-mediated generation and genetic correction of disease-specific human induced pluripotent stem cells." (url). Current Gene Therapy. 14: 461–472. doi:10.2174/1566523214666140918101725. Retrieved November 11, 2014.
- ↑ Dupuy, A. (2013). "Targeted gene therapy of xeroderma pigmentosum cells using meganuclease and TALEN™". PLOS ONE. 8 (11): e78678. doi:10.1371/journal.pone.0078678. PMC 3827243
. PMID 24236034.
- ↑ Osborn, M. J. (2013). "TALEN-based gene correction for epidermolysis bullosa" (url). Molecular Therapy. 21 (6): 1151–1159. doi:10.1038/mt.2013.56. Retrieved November 11, 2014.
- ↑ Valton, Julien; Guyot, Valérie; Marechal, Alan; Filhol, Jean-Marie; Juillerat, Alexandre; Duclert, Aymeric; Duchateau, Philippe; Poirot, Laurent (2015-09-01). "A Multidrug-resistant Engineered CAR T Cell for Allogeneic Combination Immunotherapy". Molecular Therapy: The Journal of the American Society of Gene Therapy. 23 (9): 1507–1518. doi:10.1038/mt.2015.104. ISSN 1525-0024. PMID 26061646.
- ↑ Poirot, Laurent; Philip, Brian; Schiffer-Mannioui, Cécile; Clerre, Diane Le; Chion-Sotinel, Isabelle; Derniame, Sophie; Bas, Cécile; Potrel, Pierrick; Lemaire, Laetitia (2015-07-16). "Multiplex genome edited T-cell manufacturing platform for "off-the-shelf" adoptive T-cell immunotherapies". Cancer Research. 75: canres.3321.2014. doi:10.1158/0008-5472.CAN-14-3321. ISSN 0008-5472. PMID 26183927.
- ↑ Boissel, Sandrine; Jarjour, Jordan; Astrakhan, Alexander; Adey, Andrew; Gouble, Agnès; Duchateau, Philippe; Shendure, Jay; Stoddard, Barry L.; Certo, Michael T. (2014-02-01). "megaTALs: a rare-cleaving nuclease architecture for therapeutic genome engineering". Nucleic Acids Research. 42 (4): 2591–2601. doi:10.1093/nar/gkt1224. ISSN 1362-4962. PMC 3936731
. PMID 24285304.
- ↑ "Pros and Cons Of ZNFS, TALENS, AND CRISPR/CAS". The Jackson Laboratory. March 2014.
- ↑ "CRISPR". www.abclawcenters.com. Retrieved 2016-09-13.
- ↑ Boglioli, Elsy; Richard, Magali. "Boston Consulting Group - Report on Gene Editing Precision" (PDF).
External links
- Cellectis
- E-TALEN.org A comprehensive tool for TALEN design
- TALengineering.org A comprehensive, publicly available resource for engineered TAL effector technology
- TALengineering newsgroup Newsgroup for discussion of engineered TAL effector technology
- www.taleffectors.com An open resource for TAL effector constructs
- PDB Molecule of the Month An entry in the Protein Database's monthly structural highlight