Future Circular Collider

The Future Circular Collider (FCC) study aims at developing conceptual designs for a post-LHC particle accelerator research infrastructure in a global context, with an energy significantly above that of previous circular colliders (SPS, Tevatron, LHC).[1][2]
The FCC study explores the feasibility of different particle collider scenarios with the aim of significantly expanding the current energy and luminosity frontiers. It aims to complement existing technical designs for linear electron/positron colliders (ILC and CLIC).
The study has an emphasis on proton/proton (hadron) and electron/positron (lepton) colliders while a hadron/lepton scenario is also examined. The study explores the potential of hadron and lepton circular colliders, performing an in-depth analysis of infrastructure and operation concepts and considering the technology research and development programmes that are required to build and operate a future circular collider. A conceptual design is planned to be delivered before the end of 2018, in time for the next update of the European Strategy for Particle Physics.
Background
The study hosted by CERN has been initiated as a direct response to the high-priority recommendation of the updated European Strategy for Particle Physics, published in 2013:
"CERN should undertake design studies for accelerator projects in a global context, with emphasis on proton-proton and electron- positron high-energy frontier machines. These design studies should be coupled to a vigorous accelerator R&D programme, including high-field magnets and high-gradient accelerating structures, in collaboration with national institutes, laboratories and universities worldwide."
This is in line with the recommendations of the United States’ Particle Physics Project Prioritization Panel (P5) and of the International Committee for Future Accelerators ICFA).
The discovery of the Higgs boson at the LHC, together with the absence so far of any phenomena beyond the Standard Model in collisions at centre of mass energies up to 8 TeV, has triggered an interest in future colliders to push the energy and precision frontiers. A future “energy frontier” collider at 100 TeV is a “discovery machine”, reaching out to so far unknown territories. "New physics" seen at such a machine could explain observations such as the prevalence of matter over antimatter and non-zero neutrino masses.
Motivation
The LHC has greatly advanced our understanding of matter and the Standard Model, however it cannot confirm every aspect of the SM nor explore other key questions about the Universe. To find out more about dark matter, the matter/antimatter asymmetry, or the origin of neutrino masses, extended energy and mass reach and higher precision are necessary.
The Future Circular Collider (FCC) study develops options for potential high-energy frontier circular colliders at CERN for the post-LHC era that will open up new horizons in the field of fundamental physics. Among other things, it plans to study dark matter, which accounts for approximately 25% of the visible universe, make precise measurements of the Higgs boson , and explore Beyond the Standard Model theories.
A Future Circular Collider could open a window for exploring the unknown 95% of the Universe: What is dark matter? Are there supersymmetric particles? Are there other fundamental interactions?
The discovery of the Higgs boson was a milestone in the long-standing effort to complete the Standard Model of Particle Physics, the theory that describes the laws governing most of the known Universe. Yet the Standard Model cannot explain several observations, such as:
- evidence for dark matter,
- prevalence of matter over antimatter,
- the neutrino masses.
Expanding our understanding of the fundamental laws of nature requires the energy frontier to be pushed further. Reaching this goal within the 21st century in an efficient and reliable way calls for a larger circular collider.
The FCC, with its high precision and high energy reach, could extend the search of new particles and interactions well beyond the LHC, that may hold the key to understanding those unexplained phenomena.

Scope
The FCC Study puts an emphasis on proton/proton high-energy and electron/positron high-intensity frontier machines. A hadron/lepton interaction scenario is also examined.
A 100 TeV hadron collider in a 100 km long tunnel defines therefore the overall infrastructure for the FCC study. The development of baseline designs for an energy-frontier hadron collider and a luminosity-frontier electron/positron collider forms the core of the study.
The FCC study will identify the technological advancements required for reaching the new energy and intensity frontiers and will make the first step by performing technology feasibility assessments. The study will provide an analysis of the infrastructure and operation cost while cost optimization, greater efficiency and reliable operation are key parameters in the study.
The FCC study explores the physics cases for all collider scenarios in a coordinated way that embraces discovery and precision physics. Scientists and engineers are working on the detector concepts needed to address the physics questions in each of the scenarios (hh, ee, he). The work programme includes experiment and detector concept studies to allow new physics to be explored. Detector technologies will be based on experiment concepts, the projected collider performances and the physics cases. Innovative technologies have to be developed in diverse fields, such as cryogenics, superconductivity, material science, and computer science, including new data processing and data management concepts.
Creativity and innovation are needed to develop the physics case, meet the required accelerator parameters and realise unprecedented experiments.
Accelerators
The FCC Study will develop and evaluate three accelerator concepts for its conceptual design report.
FCC-hh (proton/proton and ion/ion)
A future energy-frontier hadron collider will be able to discover force carriers of new interactions up to masses of around 30 TeV. In such a machine, the discovery reach for dark matter particles will extend well beyond the TeV region, while supersymmetric partners of quarks and gluons can be produced at masses up to 15-20 TeV and the search for a possible substructure inside quarks can be extended down to distance scales of 10−21 m. Billions of Higgs bosons and trillions of top quarks will be produced, creating new opportunities for the study of rare decays and flavour physics, which tremendously benefit from higher collision energies.
A hadron collider will also extend the study of Higgs and gauge boson interactions to energies well above the TeV scale, providing a way to analyse in detail the mechanism underlying the breaking of the electroweak symmetry.
The FCC-hh collider offers an opportunity to push the exploration of the collective structure of matter at the most extreme density and temperature conditions to new frontiers through the study of heavy-ion collisions.[3][4]
FCC-ee (electron/positron)
A lepton collider with centre-of-mass collision energies between 90 and 350 GeV is considered a potential intermediate step towards the realisation of the hadron facility. Clean experimental conditions have given e+e− storage rings a strong record of accomplishment both for measuring known particles with the highest precision and for exploring the unknown.
More specifically, high luminosity and improved handling of lepton beams would create the opportunity to measure the properties of the Z, W, Higgs, and top particles, as well as the strong interaction, with unequalled accuracy.[5][6]
The unique measurements of invisible or exotic decays of the Higgs and Z bosons would offer discovery potential for dark matter or heavy neutrinos. In effect, the FCC-ee could enable profound investigations of electroweak symmetry breaking and open a broad indirect search for new physics over several orders of magnitude in energy or couplings.
FCC-he (electron/proton)
With the huge energy provided by the 50 TeV proton beam and the potential availability of an electron beam with energy of the order of 60 GeV, new horizons open up for the physics of deep inelastic electron/proton scattering. The FCC-he collider would be both a high-precision Higgs factory and a powerful microscope that could discover new particles, study quark/gluon interactions, and examine possible further substructure of matter in the world. With such a programme, accompanied by unprecedented measurements of strong and electroweak interaction phenomena, the hadron/electron collider is another unique complement to the exploration of nature at high energies.
Technologies
As the development of a next generation particle accelerator requires state-of-the-art technology, the FCC Study has undertaken extensive R&D to create the equipment and machines that are needed for the realization of the project. Technology R&D relies on interdisciplinary synergies, taking into account the experience from past and present accelerator projects.[7]

The foundations for these advancements are being laid in a focused R&D programmes:
- a 16 Tesla high-field accelerator magnet and related super-conductor research,
- a 100 MW radiofrequency acceleration system that can efficiently transfer power from the electricity grid to the beams,
- a highly efficient large-scale cryogenics infrastructure to cool down superconducting accelerator components and the accompanying refrigeration systems.

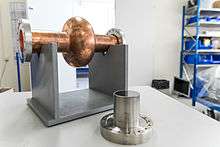
Numerous other technologies are needed for reliable, sustainable and efficient operation. The effective interplay of different science and technology domains (accelerator physics, high-field magnets, cryogenics, vacuum, civil engineering, material science, superconductors) is the key to success.
Advancing technology and engineering for high-energy particle physics and accelerators creates significant benefits for society. These innovations lead to new products and services that change our daily lives.
The rich and diverse FCC R&D programme attracts significant interest from students of different fields ranging from material sciences, chemistry, mechanical and electrical engineering, over computer science, mathematics, reliability engineering to technology management, international business management, entrepreneurship, patent and innovation management, international law and social sciences.
Magnet Technologies
High-field superconducting magnets are a key enabling technology for a frontier hadron collider. To steer a 50 TeV beam over a 100 km tunnel, 16-Tesla dipoles will be necessary, twice the strength of the magnetic field of the LHC.

The magnet R&D aims to extend the range of operation of accelerator magnets based on Low Temperature Superconductors (LTS) up to 16 T and explore the technological challenges inherent to the use of High Temperature Superconductors (HTS) for accelerator magnets in the 20 T range.
Superconducting Radiofrequency Cavities
The beams that move in a circular accelerator lose a percentage of their energy, approximately 4.4%, at every turn. To maintain their energy, a system of radiofrequency constantly provides 50MW to each beam.
At a 45 GeV system, the percentage of energy loss is expected to increase and the superconducting RF cavities will have to deliver a total of 100 MW of acceleration power.
Cryogenics
Liquefaction of gas is a power-intensive operation that requires state-of-the-art cryogenic technology. The future lepton and the hadron colliders would make intensive use of low-temperature superconducting devices, operated at 4.5 K and 1.8 K, requiring very large-scale distribution, recovery, and storage of cryogenic fluids.

As a result, the cryogenic systems that have to be developed correspond to two to four times the presently deployed systems and require increased availability and maximum energy efficiency. Any further improvements in cryogenics are expected to find wide applications in medical imaging techniques.
The cryogenic beam vacuum system for an energy-frontier hadron collider must absorb an energy of 50 W per meter at cryogenic temperatures. To protect the magnet cold bore from the head load, the vacuum system needs to be resistant against electron cloud effects, highly robust, and stable under superconducting quench conditions.
It should also allow fast feedback in presence of impedance effects. New composite materials have to be developed to achieve these unique thermo-mechanics and electric properties for collimation systems. Such materials could also be complemented with the on-going exploration of thin-film NEG coating that is used in the internal surface of the copper vacuum chambers.
Collimation
Finally, a 100 TeV hadron collider requires efficient and robust collimators, as 100 kW of hadronic background is expected at the interaction points. Moreover, fast self-adapting control systems with sub-millimeter collimation gaps are necessary to prevent irreversible damage of the machine and manage the 180 GJ stored in each magnet.
To address these challenges, the FCC Study searches for designs that can withstand the large energy loads with acceptable transient deformation and no permanent damage. Novel composites with unprecedented thermo-mechanical and electric properties will be investigated in cooperation with the FP7 HiLumi LHC DS and EuCARD2 programmes.
Timescale
The Large Hadron Collider at CERN with its High Luminosity upgrade is the world’s primary instrument for exploring the energy frontier until 2035. This defines the time window for preparing a post-LHC high-energy physics research infrastructure.
LEP and LHC have shown that a time-frame of 30 years is appropriate for the design and construction of a large accelerator complex and particle detectors. The significant lead time calls for a coordinated global effort. The goal is to ensure the seamless continuation of the world’s particle physics programme after the LHC era.

International Collaboration
The FCC study hosted by CERN is an international collaboration of more than 70 institutes from all over the world.

This prepares the ground for geographically well-balanced contributions, leveraging the competences of world experts in the numerous areas concerned. It also ensures that the entire worldwide scientific community is involved from the very start of the endeavour.
Bringing together physics, experiments, accelerator concepts and technology R&D within a single study will result in a coherent and consistent design for a future large-scale research infrastructure.
The experience from the operation of LEP and LHC and the unique opportunity to test the novel technologies in the High Luminosity LHC provide a solid basis for assessing the feasibility of a post-LHC particle accelerator. The study will deliver a Conceptual Design Report (CDR) by the end of 2018, in time for the next European Strategy for Particle Physics.
Organisation
The FCC Study is hosted by CERN, also a Participant in the Collaboration, as a direct response to the recommendation made in the update of the European Strategy for Particle Physics 2013, adopted by the Organisation's Council. The Study is governed by three bodies: the International Collaboration Board (ICB), the International Steering Committee (ISC), and the International Advisory Committee (IAC).

The ICB reviews the resource needs of the Study and finds matches within the Collaboration. It so channels the contributions from the Participants of the Collaboration aiming at a geographically well-balanced and topically complementary network of contributions The ISC is the supervisory and main governing body for the execution of the Study and acts on behalf of the Collaboration.
It is responsible for the proper execution and implementation of the decisions of the ICB, deriving and formulating the strategic scope, individual goals and the work programme of the Study. Its work is facilitated by the Coordination Group, the main executive body of the project, which coordinates the individual work packages and performs the day-to-day management of the Study.
Finally, the IAC reviews the scientific and technical progress of the Study and shall submit scientific and technical recommendations to the International Steering Committee to assist and facilitate major technical decisions.
Similar studies
Following the first run of the LHC, there is consensus in the scientific community that the results will have to be complemented by a collider that can study the discoveries in greater detail by producing different kinds of collisions. Several studies for future particle accelerators, both linear and circular, are therefore carried out.
The Compact Linear Collider (CLIC) examines the feasibility of a high-energy (up to 3 TeV), high-luminosity lepton (electron/positron) collider, while the International Linear Collider is a similar project, planned to have a collision energy of 500 GeV. In 2013, the two studies formed an organisational partnership, the Linear Collider Collaboration (LCC) to coordinate and advance the global development work for the linear collider. Concerning the LHC, a high-luminosity upgrade is planned to extend its operation lifetime into the mid-2030s. The upgrade will facilitate the detection of rare processes and improve statistically marginal measurements.
References
- ↑ Michael Benedikt, Frank Zimmermann. "Future Circular Collider (FCC) Study". Retrieved 2014-03-28.
- ↑ Michael Benedikt, Frank Zimmermann. "Future Circular Collider (FCC) Study". Retrieved 2015-09-21.
- ↑ Zimmerman, F.; et al. "Challenges for Highest Energy Circular Colliders" (PDF).
- ↑ "Luminosity goals for a 100-TeV pp".
- ↑ John Ellis, Tevong You. "Sensitivities of Prospective Future e+e- Colliders to Decoupled New Physics". arXiv:1510.04561
.
- ↑ David d'Enterria. "Physics case of FCC-ee". arXiv:1601.06640
.
- ↑ "Future Hadron Colliders: from physics perspectives to technology R&D".